Teste dein Wissen zum Thema Michelson-Interferometer!
Michelson-interferometer.
Was es mit dem Michelson-Interferometer auf sich hat und wieso es heute noch von besonderer Bedeutung ist erfährst du hier.
Schau dir auf jeden Fall noch das Video zum Artikel an. Darin sind alle relevanten Inhalte für dich audiovisuell aufbereitet.

Michelson-Interferometer einfach erklärt
Michelson-interferometer funktion, michelson-interferometer interferenzringe, michelson-interferometer wegmessung, michelson-interferometer als spektrometer, michelson-interferometer brechungsindex, michelson-interferometer wellenlänge, michelson-morley-experiment.
Der Michelson-Interferometer ist ein weit verbreiteter Aufbau für optische Experimente. Mit ihm wird optische Interferometrie betrieben. Das sind Messmethoden, die die Überlagerung oder Interferenz von Wellen zur Bestimmung bestimmter Größen ausnutzen.
Der Aufbau besteht aus verschiedenen Komponenten, mit denen du einen Laserstrahl beeinflusst. Mit Hilfe eines Strahlteilers , teilst du den eingehenden Laserstrahl in zwei separate Arme auf. Diese beiden Arme werden dann an Spiegeln zurück zum Strahlteiler reflektiert. Aufgrund des Superpositionsprinzips , erzeugt die Überlagerung der beiden Strahlen einen resultierenden Strahl. Anschließend wird der resultierende Strahl auf eine Diode oder Kamera ausgelenkt. Mit dieser nimmt man das Interferenzmuster des Strahls auf.
Abhängig von der Messung die du machst, variierst du die Ganglänge der beiden Strahlen oder bringst weitere Medien in die Strahlengänge ein.
Ein Interferometer nutzt die Interferenz von Wellen für Präzisionsmessungen aus. Das machst du möglich, indem du eine Lichtwelle in zwei Teile aufteilst. Diese zwei Wellen durchlaufen dann mit unterschiedlicher Laufzeit verschieden Lange strecken. Dabei kommt es zu einer Phasenverschiebung und beide Wellen interferieren beim erneuten aufeinander Treffen miteinander. Hierbei werden alle Eigenschaften gemessen, die die Weglänge der Wellen und somit die Eigenschaften der resultierenden Welle ändern. Das ist zum Beispiel die Änderung der Länge einer der beiden Strahlengänge zur Längenmessung oder die Variation des Brechungsindex zur Bestimmung von Materialeigenschaften. Die resultierende, überlagerte Welle nimmst du mit einer Art Schirm oder elektronischem Detektor auf.
Auch beim Michelson – Interferometer wird Laserlicht in zwei Strahlen aufgeteilt. Du teilst die Lichtwelle mithilfe eines halbdurchlässigen Spiegels in zwei Lichtwellen auf. Dieser Spiegel ist so beschaffen, dass ein Teil des Lichts durchgelassen wird, ein anderer Teil jedoch um 90° reflektiert wird. Diese beiden Strahlen treffen am Ende ihrer jeweiligen Strahlengänge auf vollständig reflektierende Spiegel. Diese werfen das Licht wieder zurück zum Strahlteiler . Erneut wird ein Teil durchgelassen und ein Teil reflektiert, wodurch es hinter dem Spiegel zur Interferenz kommt.
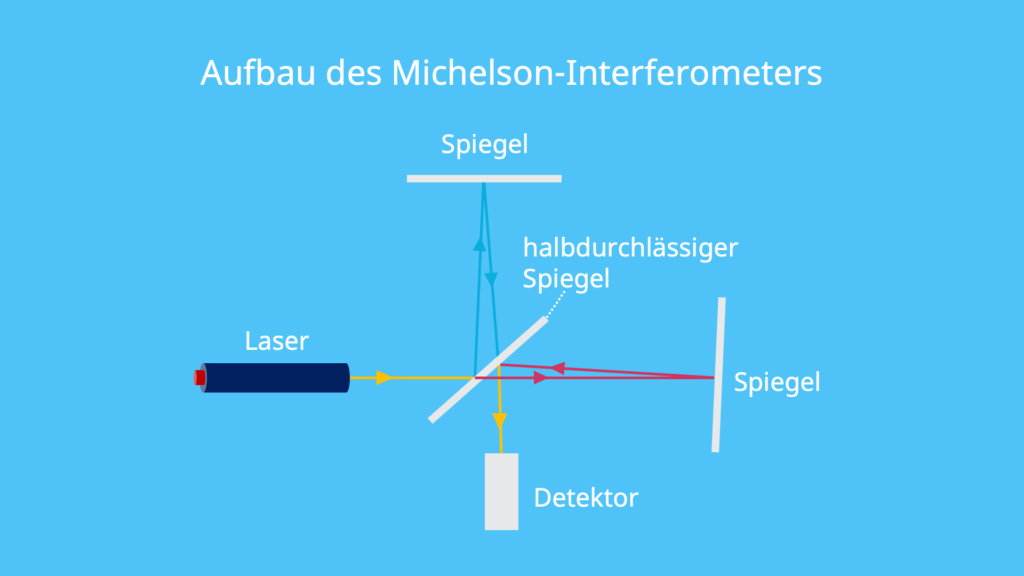
Durch Veränderung der optischen Weglänge eine der beiden Lichtwellen, wird dessen Phase verändert. Sind damit beide Wellen in Phase , so addieren sich deren Amplituden und es kommt zur konstruktiven Interferenz . Sind sie gegenphasig , löschen sich beide Lichtwellen gegenseitig aus und es kommt zur destruktiven Interferenz .
Damit du dies an deinem Michelson – Interferometer feststellst, misst du an deinem Detektor die Intensität des resultierenden Strahls.

Diesen Weg bestimmst du dann über den folgenden Zusammenhang:

Bei Betrachtung einer ebenen Welle erscheint bei konstruktiver Interferenz ein heller Fleck, bei destruktiver Interferenz ein dunkler.
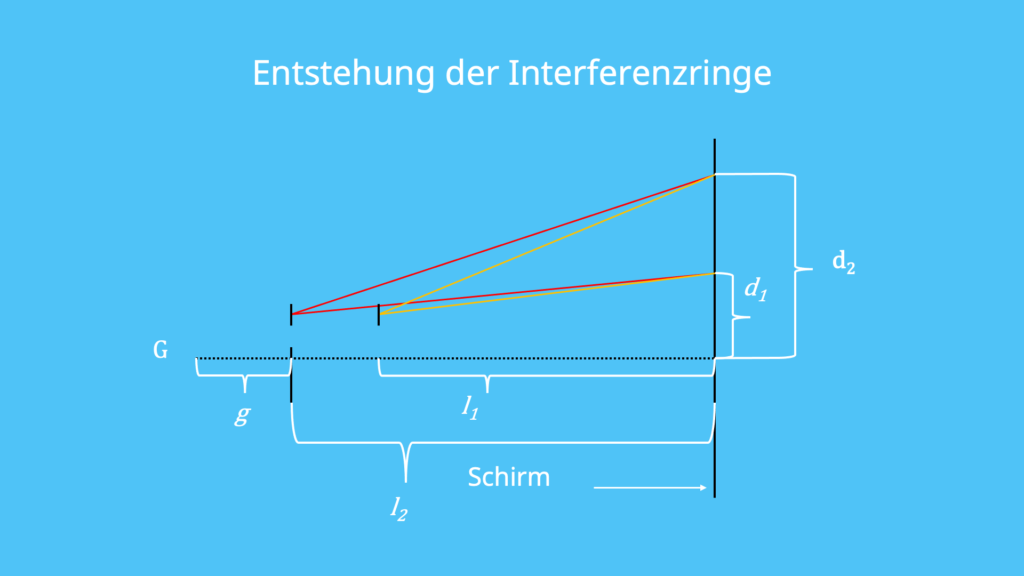
Das Michelson – Interferometer ist dafür geeignet, um langsame Änderungen der Weglängendifferenz der beiden Teilstrahlen zu messen. Die hierbei erreichte Auflösung liegt bei einer halben Wellenlänge des verwendeten Lichts. Verwendest du also einen Laser so entspricht das einigen hundert Nanometern.
Willst du die Weglängendifferenz messen, verschiebst du einen der beiden undurchlässigen Spiegel und zählst dabei die durchlaufenen Interferenzmaxima beziehungsweise Minima . Um zu sehen was deine Maxima oder Minima sind zählst du also die hellen oder dunklen Ringe deines Interferenzmusters .
Jedes Minimum beziehungsweise Maximum entspricht dann einer Weglängenänderung von einer halben Wellenlänge . Das heißt die Position des Spiegels hat sich um eine halbe Wellenlänge verschoben. Jedoch ist es dir nicht möglich die absolute Weglänge, absolute Weglängendifferenz oder die Richtung deiner Bewegung zu messen.
Um mit dem Michelson – Interferometer Spektroskopie zu betreiben, musst du den Aufbau nur geringfügig ändern. Du brauchst zunächst eine breitbandige Infrarot Quelle, also eine Quelle für infrarotes Licht . Vor dem Detektor befestigst du ein durchlässiges Gefäß, welches du mit deiner, zu untersuchenden, Substanz füllst. Nun durchläufst du mit deinem Spiegel mehrere Wellenlängen und misst die Anzahl der destruktiven- und konstruktiven Interferenzen. Mit einer Fourier – Transformation erhältst du daraus dein Spektrum und somit die Identität deiner Substanz.
Der Michelson – Interferometer ermöglicht dir auch die Messung des Brechungsindexes eines Gases. Diesmal bleiben die Spiegel fest. In einen der beiden Teilstrahlen bringst du ein gasgefülltes Gefäß ein. Mit einer Pumpe, variierst du den Gasdruck darin und somit die Anzahl der Moleküle innerhalb des Gefäßes.
Zwischen Gasdruck und Brechungsindex besteht ein linearer Zusammenhang:

Der Michelson-Interferometer wurde das erste Mal zwischen April und Juli 1887 im berühmten Michelson-Morley-Experiment angewandt. Die beiden Wissenschaftler Albert A. Michelson und Edward W. Morley waren die Experimentatoren und Namensgeber des Experiments. Ihr Ziel war es, den Äther nachzuweisen. Im ausgehenden 17. Jahrhundert wurde generell angenommen, dass elektromagnetische Wellen , ähnlich wie Schallwellen , ein Medium brauchen um sich auszubreiten. Für Schallwellen ist das in den meisten Fällen die Luft und für elektromagnetische Wellen sollte das der Äther sein.
Mithilfe des Interferometers sollten die sogenannten Ätherwinde nachgewiesen werden. Aufgrund der Bewegung der Erde durch diesen Äther würde der Lichtstrahl in Bewegungsrichtung einen längeren Strahlengang haben als der Strahl senkrecht dazu. So wollten die beiden Wissenschaftler die Relativbewegung des Äthers zur Erde messen.
Das Ergebnis kam negativer heraus, was für viele Wissenschaftler ein Mysterium schuf, das sie nicht klären konnten. Erst durch die Formulierung der speziellen Relativitätstheorie konnte es gelöst werden. Aufgrund seiner Signifikanz wird das Michelson-Morley-Experiment auch als ein Schlüsselexperiment hin zur Entwicklung der Relativitätstheorie betrachtet.
Beliebte Inhalte aus dem Bereich Optik & Akustik
- Kohärenz (Physik) Dauer: 03:48
- Frequenz berechnen Dauer: 04:11
- Additive Farbmischung und subtraktive Farbmischung Dauer: 05:14
Weitere Inhalte: Optik & Akustik
Hallo, leider nutzt du einen AdBlocker.
Auf Studyflix bieten wir dir kostenlos hochwertige Bildung an. Dies können wir nur durch die Unterstützung unserer Werbepartner tun.
Schalte bitte deinen Adblocker für Studyflix aus oder füge uns zu deinen Ausnahmen hinzu. Das tut dir nicht weh und hilft uns weiter.
Danke! Dein Studyflix-Team
Wenn du nicht weißt, wie du deinen Adblocker deaktivierst oder Studyflix zu den Ausnahmen hinzufügst, findest du hier eine kurze Anleitung . Bitte lade anschließend die Seite neu .

- Why Does Water Expand When It Freezes
- Gold Foil Experiment
- Faraday Cage
- Oil Drop Experiment
- Magnetic Monopole
- Why Do Fireflies Light Up
- Types of Blood Cells With Their Structure, and Functions
- The Main Parts of a Plant With Their Functions
- Parts of a Flower With Their Structure and Functions
- Parts of a Leaf With Their Structure and Functions
- Why Does Ice Float on Water
- Why Does Oil Float on Water
- How Do Clouds Form
- What Causes Lightning
- How are Diamonds Made
- Types of Meteorites
- Types of Volcanoes
- Types of Rocks
Michelson-Morley Experiment
What is michelson-morley experiment.
About 150 years ago, physicists believed that light waves require a medium to pass through. The Michelson-Morley experiment was performed by American scientists Albert Michelson and Edward Morley between April and July 1887. The purpose of this experiment is to prove the existence of ether. This hypothetical medium permeating space was thought to be the carrier of light waves. This experiment aimed to measure the speed of light in two perpendicular directions relative to the ether. The result was negative. Michelson and Morley did not find any significant difference between the speed of light in the direction of the presumed ether and the speed at right angles.

Experimental Arrangement of Michelson-Morley Experiment
To the effect of ether on the speed of light, Michelson designed a device now known as an interferometer. It is a sensitive optical device that compares the optical path lengths for light moving in two mutually perpendicular directions and utilized the interference of light waves to perform measurements of incredible accuracy. It sent a beam of light from a single source through a half-silvered mirror (beam splitter) that split it into two beams traveling perpendicular to each other. After departing the splitter, the beams traveled out to the long arms of the interferometer, where they were reflected in the middle by two small mirrors. Then, they recombined on the far side of the splitter, producing a pattern of constructive and destructive interference. The pattern of fringes, as observed by an eyepiece, is based on the length of the arms. Any slight change in the length would alter the amount of time the beams spent in transit. This change would then be observed as a shift in the positions of the interference fringes.

Conclusion of Michelson-Morley Experiment
Michelson and Morley measured the speed of light by observing the interference fringes produced by the two beams. They expected that the light would travel faster along an arm if oriented in the same direction as the ether was moving, and slower if oriented in the opposite direction. Since the two arms were perpendicular, there is only one way that light would travel at the same speed in both arms and arrive simultaneously at the eyepiece. It was possible if the instrument were motionless to the ether. If this were not the case, the crests and troughs of the light waves in the two arms would arrive and interfere slightly out of synchronization, thus reducing the intensity. Although Michelson and Morley expected different speeds of light in each direction, they found no noticeable shift in the fringes. Otherwise, that would indicate a different speed in any orientation or at any position of the Earth in its orbit. This null result seriously discredited existing ether theories. Eventually, it led to the proposal by Albert Einstein in 1905 that the speed of light is a universal constant.
Article was last reviewed on Saturday, May 9, 2020
Related articles

One response to “Michelson-Morley Experiment”
If the above experiment (as well as Airy’s Failure) did not detect the earth’s movement, how come Focault’s pendulum does? Can’t prove truth via lies.
Leave a Reply Cancel reply
Your email address will not be published. Required fields are marked *
Save my name, email, and website in this browser for the next time I comment.
Popular Articles
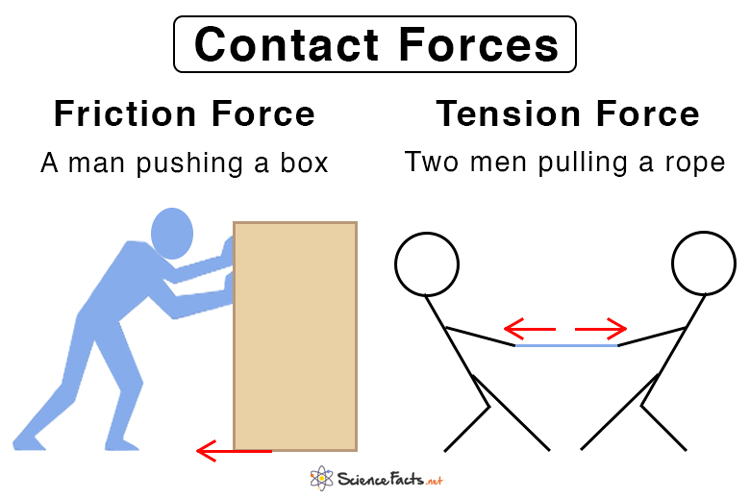
Join our Newsletter
Fill your E-mail Address
Related Worksheets
- Privacy Policy
© 2024 ( Science Facts ). All rights reserved. Reproduction in whole or in part without permission is prohibited.
previous home next Spanish
The Michelson-Morley Experiment
Michael Fowler, University of Virginia
The Nature of Light
As a result of Michelson’s efforts in 1879, the speed of light was known to be 186,350 miles per second with a likely error of around 30 miles per second. This measurement, made by timing a flash of light travelling between mirrors in Annapolis, agreed well with less direct measurements based on astronomical observations. Still, this did not really clarify the nature of light. Two hundred years earlier, Newton had suggested that light consists of tiny particles generated in a hot object, which spray out at very high speed, bounce off other objects, and are detected by our eyes. Newton’s arch-enemy Robert Hooke, on the other hand, thought that light must be a kind of wave motion , like sound. To appreciate his point of view, let us briefly review the nature of sound.
The Wavelike Nature of Sound
Actually, sound was already quite well understood by the ancient Greeks. The essential point they had realized is that sound is generated by a vibrating material object, such as a bell, a string or a drumhead. Their explanation was that the vibrating drumhead, for example, alternately pushes and pulls on the air directly above it, sending out waves of compression and decompression (known as rarefaction), like the expanding circles of ripples from a disturbance on the surface of a pond. On reaching the ear, these waves push and pull on the eardrum with the same frequency (that is to say, the same number of pushes per second) as the original source was vibrating at, and nerves transmit from the ear to the brain both the intensity (loudness) and frequency (pitch) of the sound.
There are a couple of special properties of sound waves (actually any waves) worth mentioning at this point. The first is called interference . This is most simply demonstrated with water waves. If you put two fingers in a tub of water, just touching the surface a foot or so apart, and vibrate them at the same rate to get two expanding circles of ripples, you will notice that where the ripples overlap there are quite complicated patterns of waves formed. The essential point is that at those places where the wave-crests from the two sources arrive at the same time, the waves will work together and the water will be very disturbed, but at points where the crest from one source arrives at the same time as the wave trough from the other source, the waves will cancel each other out, and the water will hardly move.
There's a standard physics lab experiment illustrating this point, it's called two-slit interference. A monochromatic plane wave approaches a screen in which there are two narrow slit openings. If the slit opening width is smaller than the wavelength, a single slit will give approximately semicircular waves coming out the other side, the disturbance radiates out from the slit. The two slits together show the pattern generated by interference. It's best seen by clicking on this applet . Watch how the pattern changes with wavelength and with slit separation.
You can hear the interference effect for sound waves by playing a constant note through stereo speakers. As you move around a room, you will hear quite large variations in the intensity of sound. Of course, reflections from walls complicate the pattern. This large variation in volume is not very noticeable when the stereo is playing music, because music is made up of many frequencies, and they change all the time. The different frequencies, or notes, have their quiet spots in the room in different places. The other point that should be mentioned is that high frequency tweeter-like sound is much more directional than low frequency woofer-like sound. It really doesn’t matter where in the room you put a low-frequency woofer—the sound seems to be all around you anyway. On the other hand, it is quite difficult to get a speaker to spread the high notes in all directions. If you listen to a cheap speaker, the high notes are loudest if the speaker is pointing right at you. A lot of effort has gone into designing tweeters, which are small speakers especially designed to broadcast high notes over a wide angle of directions.

Is Light a Wave?
Bearing in mind the above minireview of the properties of waves, let us now reconsider the question of whether light consists of a stream of particles or is some kind of wave. The strongest argument for a particle picture is that light travels in straight lines. You can hear around a corner, at least to some extent, but you certainly can’t see. Furthermore, no wave-like interference effects are very evident for light. Finally, it was long known, as we have mentioned, that sound waves were compressional waves in air. If light is a wave, just what is waving? It clearly isn’t just air, because light reaches us from the sun, and indeed from stars, and we know the air doesn’t stretch that far, or the planets would long ago have been slowed down by air resistance.
Despite all these objections, it was established around 1800 that light is in fact some kind of wave. The reason this fact had gone undetected for so long was that the wavelength is really short, about one fifty-thousandth of an inch. In contrast, the shortest wavelength sound detectable by humans has a wavelength of about half an inch. The fact that light travels in straight lines is in accord with observations on sound that the higher the frequency (and shorter the wavelength) the greater the tendency to go in straight lines. Similarly, the interference patterns mentioned above for sound waves or ripples on a pond vary over distances of the same sort of size as the wavelengths involved. Patterns like that would not normally be noticeable for light because they would be on such a tiny scale. In fact, it turns out, there are ways to see interference effects with light. A familiar example is the many colors often visible in a soap bubble. These come about because looking at a soap bubble you see light reflected from both sides of a very thin film of water—a thickness that turns out to be comparable to the wavelength of light. The light reflected from the lower boundary has to go a little further to reach your eye, so that light wave must wave an extra time or two before getting to your eye compared with the light reflected from the top of the film. What you actually see is the sum of the light reflected from the top and that reflected from the bottom. Thinking of this now as the sum of two sets of waves, the light will be bright if the crests of the two waves arrive together, dim if the crests of waves reflected from the top layer arrive simultaneously with the troughs of waves reflected from the bottom layer. Which of these two possibilities actually occurs for reflection from a particular bit of the soap film depends on just how much further the light reflected from the lower surface has to travel to reach your eye compared with light from the upper surface, and that depends on the angle of reflection and the thickness of the film. Suppose now we shine white light on the bubble. White light is made up of all the colors of the rainbow, and these different colors have different wavelengths, so we see colors reflected, because for a particular film, at a particular angle, some colors will be reflected brightly (the crests will arrive together), some dimly, and we will see the ones that win.
If Light is a Wave, What is Waving?
Having established that light is a wave, though, we still haven’t answered one of the major objections raised above. Just what is waving? We discussed sound waves as waves of compression in air. Actually, that is only one case—sound will also travel through liquids, like water, and solids, like a steel bar. It is found experimentally that, other things being equal, sound travels faster through a medium that is harder to compress: the material just springs back faster and the wave moves through more rapidly. For media of equal springiness, the sound goes faster through the less heavy medium, essentially because the same amount of springiness can push things along faster in a lighter material. So when a sound wave passes, the material—air, water or solid—waves as it goes through. Taking this as a hint, it was natural to suppose that light must be just waves in some mysterious material, which was called the aether , surrounding and permeating everything. This aether must also fill all of space, out to the stars, because we can see them, so the medium must be there to carry the light. (We could never hear an explosion on the moon, however loud, because there is no air to carry the sound to us.) Let us think a bit about what properties this aether must have. Since light travels so fast, it must be very light, and very hard to compress. Yet, as mentioned above, it must allow solid bodies to pass through it freely, without aether resistance, or the planets would be slowing down. Thus we can picture it as a kind of ghostly wind blowing through the earth. But how can we prove any of this? Can we detect it?
Detecting the Aether Wind: the Michelson-Morley Experiment
Detecting the aether wind was the next challenge Michelson set himself after his triumph in measuring the speed of light so accurately. Naturally, something that allows solid bodies to pass through it freely is a little hard to get a grip on. But Michelson realized that, just as the speed of sound is relative to the air, so the speed of light must be relative to the aether. This must mean, if you could measure the speed of light accurately enough, you could measure the speed of light travelling upwind, and compare it with the speed of light travelling downwind, and the difference of the two measurements should be twice the windspeed. Unfortunately, it wasn’t that easy. All the recent accurate measurements had used light travelling to a distant mirror and coming back, so if there was an aether wind along the direction between the mirrors, it would have opposite effects on the two parts of the measurement, leaving a very small overall effect. There was no technically feasible way to do a one-way determination of the speed of light.
At this point, Michelson had a very clever idea for detecting the aether wind. As he explained to his children (according to his daughter), it was based on the following puzzle:
Suppose we have a river of width w (say, 100 feet), and two swimmers who both swim at the same speed v feet per second (say, 5 feet per second). The river is flowing at a steady rate, say 3 feet per second. The swimmers race in the following way: they both start at the same point on one bank. One swims directly across the river to the closest point on the opposite bank, then turns around and swims back. The other stays on one side of the river, swimming upstream a distance (measured along the bank) exactly equal to the width of the river, then swims back to the start. Who wins?
Let’s consider first the swimmer going upstream and back. Going 100 feet upstream, the speed relative to the bank is only 2 feet per second, so that takes 50 seconds. Coming back, the speed is 8 feet per second, so it takes 12.5 seconds, for a total time of 62.5 seconds.
Figure 1: In time t , the swimmer has moved ct relative to the water, and been carried downstream a distance vt .
The swimmer going across the flow is trickier. It won’t do simply to aim directly for the opposite bank-the flow will carry the swimmer downstream. To succeed in going directly across, the swimmer must actually aim upstream at the correct angle (of course, a real swimmer would do this automatically). Thus, the swimmer is going at 5 feet per second, at an angle, relative to the river, and being carried downstream at a rate of 3 feet per second. If the angle is correctly chosen so that the net movement is directly across, in one second the swimmer must have moved four feet across: the distances covered in one second will form a 3,4,5 triangle. So, at a crossing rate of 4 feet per second, the swimmer gets across in 25 seconds, and back in the same time, for a total time of 50 seconds. The cross-stream swimmer wins. This turns out to true whatever their swimming speed. (Of course, the race is only possible if they can swim faster than the current!)
Figure 2: This diagram is from the original paper. The source of light is at s , the 45 degree line is the half-silvered mirror, b and c are mirrors and d the observer.
Michelson’s great idea was to construct an exactly similar race for pulses of light, with the aether wind playing the part of the river. The scheme of the experiment is as follows: a pulse of light is directed at an angle of 45 degrees at a half-silvered, half transparent mirror, so that half the pulse goes on through the glass, half is reflected. These two half-pulses are the two swimmers. They both go on to distant mirrors which reflect them back to the half-silvered mirror. At this point, they are again half reflected and half transmitted, but a telescope is placed behind the half-silvered mirror as shown in the figure so that half of each half-pulse will arrive in this telescope. Now, if there is an aether wind blowing, someone looking through the telescope should see the halves of the two half-pulses to arrive at slightly different times, since one would have gone more upstream and back, one more across stream in general. To maximize the effect, the whole apparatus, including the distant mirrors, was placed on a large turntable so it could be swung around.
We have an animation, including the aether wind and the rotating turntable, here !
Let us think about what kind of time delay we expect to find between the arrival of the two half-pulses of light. Taking the speed of light to be c miles per second relative to the aether, and the aether to be flowing at v miles per second through the laboratory, to go a distance w miles upstream will take w / ( c − v ) seconds, then to come back will take w / ( c + v ) seconds. The total roundtrip time upstream and downstream is the sum of these, which works out to be 2 w c / ( c 2 − v 2 ) , which can also be written
t upstream + downstream = 2 w c ⋅ 1 1 − ( v 2 / c 2 ) .
Now, we can safely assume the speed of the aether is much less than the speed of light, otherwise it would have been noticed long ago, for example in timing of eclipses of Jupiter’s satellites. This means v 2 / c 2 is a very small number, and we can use some handy mathematical facts to make the algebra a bit easier. First, if x is very small compared to 1 , 1 / ( 1 − x ) is very close to 1 + x . (You can check it with your calculator.) Another fact we shall need in a minute is that for small x , the square root of 1 + x is very close to 1 + x / 2.
Putting all this together,
t upstream + downstream ≅ 2 w c × ( 1 + v 2 c 2 ) .
Figure 3 This is also from the original paper, and shows the expected path of light relative to the aether with an aether wind blowing.
Now, what about the cross-stream time? The actual cross-stream speed must be figured out as in the example above using a right-angled triangle, with the hypoteneuse equal to the speed c , the shortest side the aether flow speed v , and the other side the cross-stream speed we need to find the time to get across. From Pythagoras’ theorem, then, the cross-stream speed is c 2 − v 2 .
Since this will be the same both ways, the roundtrip cross-stream time will be
t cross and back = 2 w c 2 − v 2 .
This can be written in the form
2 w c 1 1 − v 2 / c 2 ≅ 2 w c 1 1 − ( v 2 / 2 c 2 ) ≅ 2 w c ( 1 + v 2 2 c 2 )
where the two successive approximations, valid for v / c = x ≪ 1 , are 1 − x ≅ 1 − ( x / 2 ) and 1 / ( 1 − x ) ≅ 1 + x .
t cross and back ≅ 2 w c × ( 1 + v 2 2 c 2 ) .
Looking at the two roundtrip times at the ends of the two paragraphs above, we see that they differ by an amount
t upstream + downstream − t cross and back ≅ 2 w c ⋅ v 2 2 c 2 .
Now, 2 w / c is just the time the light would take if there were no aether wind at all, say, a few millionths of a second. If we take the aether windspeed to be equal to the earth’s speed in orbit, for example, v / c is about 1/10,000, so v 2 / c 2 is about 1/100,000,000.
This means the time delay between the pulses reflected from the different mirrors reaching the telescope is about one-hundred-millionth of a few millionths of a second.
It seems completely hopeless that such a short time delay could be detected. However, this turns out not to be the case, and Michelson was the first to figure out how to do it. The trick is to use the interference properties of the light waves. Instead of sending pulses of light, as we discussed above, Michelson sent in a steady beam of light of a single color. This can be visualized as a sequence of ingoing waves, with a wavelength one fifty-thousandth of an inch or so. Now this sequence of waves is split into two, and reflected as previously described. One set of waves goes upstream and downstream, the other goes across stream and back. Finally, they come together into the telescope and the eye. If the one that took longer is half a wavelength behind, its troughs will be on top of the crests of the first wave, they will cancel, and nothing will be seen. If the delay is less than that, there will still be some dimming. However, slight errors in the placement of the mirrors would have the same effect. This is one reason why the apparatus is built to be rotated—see the animation ! On turning it through 90 degrees, the upstream-downstream and the cross-stream waves change places. Now the other one should be behind. Thus, if there is an aether wind, if you watch through the telescope while you rotate the turntable, you should expect to see variations in the brightness of the incoming light.
To magnify the time difference between the two paths, in the actual experiment the light was reflected backwards and forwards several times, like a several lap race.
Michelson calculated that an aether windspeed of only one or two miles a second would have observable effects in this experiment, so if the aether windspeed was comparable to the earth’s speed in orbit around the sun, it would be easy to see. In fact, nothing was observed. The light intensity did not vary at all. Some time later, the experiment was redesigned so that an aether wind caused by the earth’s daily rotation could be detected. Again, nothing was seen. Finally, Michelson wondered if the aether was somehow getting stuck to the earth, like the air in a below-decks cabin on a ship, so he redid the experiment on top of a high mountain in California. Again, no aether wind was observed. It was difficult to believe that the aether in the immediate vicinity of the earth was stuck to it and moving with it, because light rays from stars would deflect as they went from the moving faraway aether to the local stuck aether.
The only possible conclusion from this series of very difficult experiments was that the whole concept of an all-pervading aether was wrong from the start. Michelson was very reluctant to think along these lines. In fact, new theoretical insight into the nature of light had arisen in the 1860’s from the brilliant theoretical work of Maxwell, who had written down a set of equations describing how electric and magnetic fields can give rise to each other. He had discovered that his equations predicted there could be waves made up of electric and magnetic fields, and the speed of these waves, deduced from electrostatic and magnetostatic experiments, was predicted to be 186,300 miles per second. This is, of course, the speed of light, so it was natural to assume light to be made up of fast-varying electric and magnetic fields.
But this led to a big problem: Maxwell’s equations predicted a definite speed for light, and it was the speed found by measurements. But what was the speed to be measured relative to? The whole point of bringing in the aether was to give a picture for light resembling the one we understand for sound, compressional waves in a medium. The speed of sound through air is measured relative to air. If the wind is blowing towards you from the source of sound, you will hear the sound sooner. If there isn’t an aether, though, this analogy doesn’t hold up. So what does light travel at 186,300 miles per second relative to?
There is another obvious possibility, which is called the emitter theory: the light travels at 186,300 miles per second relative to the source of the light. The analogy here is between light emitted by a source and bullets emitted by a machine gun. The bullets come out at a definite speed (the muzzle velocity) relative to the barrel of the gun. If the gun is mounted on the front of a tank, which is moving forward, and the gun is pointing forward, then relative to the ground the bullets are moving faster than they would if shot from a tank at rest. The simplest way to test the emitter theory of light, then, is to measure the speed of light emitted in the forward direction by a flashlight moving in the forward direction, and see if it exceeds the known speed of light by an amount equal to the speed of the flashlight. Actually, this kind of direct test of the emitter theory only became experimentally feasible in the nineteen-sixties. It is now possible to produce particles, called neutral pions, which decay each one in a little explosion, emitting a flash of light. It is also possible to have these pions moving forward at 185,000 miles per second when they self destruct, and to catch the light emitted in the forward direction, and clock its speed. It is found that, despite the expected boost from being emitted by a very fast source, the light from the little explosions is going forward at the usual speed of 186,300 miles per second. In the last century, the emitter theory was rejected because it was thought the appearance of certain astronomical phenomena, such as double stars, where two stars rotate around each other, would be affected. Those arguments have since been criticized, but the pion test is unambiguous. The definitive experiment was carried out by Alvager et al., Physics Letters 12 , 260 (1964).
We have to mention here that the most spectacular realization (so far) of Michelson's interference techniques is the successful detection (first in 2016) of gravitational waves by a scaled-up version of the interferometer. The basic idea is that as a (transverse, polarized) gravitational wave passes the two arms have their lengths slightly altered by different amounts. The tiny effect is made detectable by having arms kilometers in length, and the beams go back and forth in the arms many times.
Einstein’s Answer
The results of the various aether detection experiments discussed above seem to leave us really stuck. Apparently light is not like sound, with a definite speed relative to some underlying medium. However, it is also not like bullets, with a definite speed relative to the source of the light. Yet when we measure its speed we always get the same result. How can all these facts be interpreted in a simple consistent way? We shall show how Einstein answered this question in the next lecture.
previous home next
- Celestial Bodies
Michelson Morley Experiment
We know a medium is absolutely necessary for any transmission in science. The medium of transmission plays an important role in efficient channeling. Waves like sound waves use air for transmission. Then, how is the light transmitted?
Let us know more about the Michelson Morley Experiment in detail to know about light transmission and velocity of the earth.
Michelson Morley Experiment was performed by two eminent scientists Albert A. Michelson and Edward W. Morley in the year 1887 to explain and demonstrate the presence of luminiferous ether.
What Is Luminiferous Ether or Aether?
Luminiferous Ether is the theoretical substance that acts as the medium for the transmission of electromagnetic waves like light rays and X-rays. Ether was assumed to be a transmission medium for the propagation of light.
Luminiferous ether was believed to be a theoretical medium in the 19th century. These substances were assumed to be frictionless, weightless and transparent substances. When the special theory of relativity was developed, the concept of luminiferous ether lost significance gradually.
Michelson Morley Experiment compared the speed of light in perpendicular directions in an attempt to detect the relative motion of matter through the stationary luminiferous aether. But this experiment yielded no results to prove a significant difference between the speed of light in the direction of movement through the presumed aether, and the speed at right angles.
The Michelson Morley Experiment was one of the failed experiments that stands as proof against the existence of the luminiferous ether concept.
Michelson and Morley tried to explain that Earth moved around the sun on its orbit, and the flow of substances like ether across the Earth’s surface could produce a detectable “ether wind”.
They tried to demonstrate the concept that the speed of the light would depend on the magnitude of the ether wind and on the direction of the beam with respect to it when the light is emitted from a source on Earth. Ether was assumed to be stationary. The idea of the experiment was to measure the speed of light in different directions in order to measure the speed of the ether relative to Earth, thus establishing its existence.
Interferometer
To measure the velocity of the Earth with the help of ether and to measure the changing pattern of the light, Albert Michelson developed a device called an interferometer.
The interferometer features the following components
- beam splitter
- beam splitter reference mirror
- coherent light source
- movable mirror
Pictorial representation of the interferometer is as shown in the figure below.
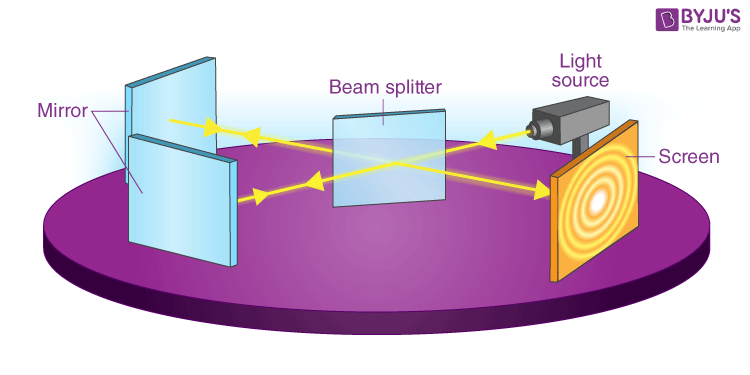
The interferometer features a half-transparent mirror that is oriented at an angle of 45°. This mirror is used to divide the light beam into two equal parts. One part of the divided beam is transmitted towards a fixed mirror and part of the divided beam is reflected in a movable mirror. The half-transparent mirror has the same effect on the returning beams, splitting them into two beams. Thus, when two diminished light beams reach the screen, a constructive and destructive wave interference pattern is observed based on the length of the arms of the device.
The speed of light was measured in the experiment by analyzing the interference fringes pattern that resulted when the light had passed through the two perpendicular arms of the interferometer. Michelson and Morley observed that light traveled faster along an arm which was oriented in the same direction as the ether. The light traveled at a slower pace in the arm oriented in the opposite direction.
As shown in the figure above, the interferometer featured perpendicular arms. The split light would travel at the same speed in both arms and therefore arrive simultaneously at the screen if the instrument were motionless with respect to the ether.
When the orientation of the interferometer is changed, the crests and troughs of the light waves produced in the two arms would interfere slightly out of synchronization.
The two scientists Michelson and Morley were expecting light to have different speeds when they travel in different directions, but they found no significantly distinguishing fringes that specified a different speed in any orientation or at any position of the Earth.
Lorentz in 1895, concluded that the Michelson Morley experiment produced the null result. Einstein wrote that If the Michelson–Morley experiment had not brought us into serious embarrassment, no one would have regarded the relativity theory as a (halfway) redemption.
When the Michelson Morley experiment was performed with increasing sophistication, the existence of ether and velocity of earth could not be proved.
Stay tuned with BYJU’S for more such interesting articles. Also, register to “BYJU’S – The Learning App” for loads of interactive, engaging Physics-related videos and an unlimited academic assist.
Related links
Frequently Asked Questions on Michelson Morley Experiment
Name the device used in michelson morley experiment..
Interferometer.
What is an interferometer?
It is a device used to measure the changing pattern of the light.
What is Luminiferous Ether?
It is the theoretical substance that acts as the medium for the transmission of electromagnetic waves.
Name the scientists who performed the experiment.
Albert A. Michelson and Edward W. Morley.
Which theory superseded the Michelson Morley Experiment?
Special theory of relativity.
Leave a Comment Cancel reply
Your Mobile number and Email id will not be published. Required fields are marked *
Request OTP on Voice Call
Post My Comment

Thank you for providing such type of articles.

Register with BYJU'S & Download Free PDFs
Register with byju's & watch live videos.

MICHELSON-MORLEY EXPERIMENT
The MICHELSON-MORLEY EXPERIMENT was performed in the basement of a WESTERN RESERVE UNIV. dormitory in July 1887 by ALBERT A. MICHELSON of the Case School of Applied Science and EDWARD W. MORLEY of Western Reserve Univ. it was designed to detect the motion of the earth through the "luminiferous aether," a theoretical substance which, according to 19th century physicists, was essential to the transmission of light.
At the heart of the experiment was an interferometer—a device invented by Michelson—which utilized the interference of light waves to perform measurements of incredible precision. Although an identical experiment undertaken by Michelson at Potsdam in 1880-81 failed to detect any motion of the earth relative to the ether, leading physicists were anxious for a repetition. Thus encouraged, Michelson and Morley first undertook a series of preliminary measurements of the velocity of light in moving fluids. After these measurements were successfully completed, the two men constructed an interferometer which was larger and more sensitive than the original Potsdam interferometer. Earlier difficulties caused by extraneous vibration were resolved when Morley designed an ingenious mounting for the device, in which the interferometer rested on a large sandstone slab which rotated while floating in a pool of mercury. Despite these improvements and the collaboration of these two great experimental scientists, the experiment again failed to detect any motion of the expected magnitude. The experiment was performed in several periods of concentrated observations between April and July 1887, in the basement of Adelbert Dormitory of WRU (later renamed Pierce Hall and then demolished in 1962).
This incongruous result puzzled the physicists of the world until 1905 when Einstein published his theory of relativity. Viewed in the light of Einstein's revolutionary work, the null results of the Michelson-Morley experiment were not only predictable, but provided experimental confirmation of Einstein's theory.
The experiment is noted by two historic markers on the south campus of Case Western Reserve University and pieces of the surviving apparatus are part of an exhibit in the Physics Department of CWRU.
Updated 25 October 2024

IMAGES
VIDEO
COMMENTS
Der Michelson-Interferometer wurde das erste Mal zwischen April und Juli 1887 im berühmten Michelson-Morley-Experiment angewandt. Die beiden Wissenschaftler Albert A. Michelson und Edward W. Morley waren die ... Schalte bitte deinen Adblocker für Studyflix aus oder füge uns zu deinen Ausnahmen hinzu. Das tut dir nicht weh und hilft uns ...
The Michelson-Morley experiment was an attempt to measure the motion of the Earth relative to the luminiferous aether, [A 1] a supposed medium permeating space that was thought to be the carrier of light waves.The experiment was performed between April and July 1887 by American physicists Albert A. Michelson and Edward W. Morley at what is now Case Western Reserve University in Cleveland ...
Michelson-Morley experiment, an attempt to detect the velocity of Earth with respect to the hypothetical luminiferous ether, a medium in space proposed to carry light waves. First performed in Germany in 1880-81 by the physicist A.A. Michelson , the test was later refined in 1887 by Michelson and Edward W. Morley in the United States .
The Michelson-Morley experiment was performed by American scientists Albert Michelson and Edward Morley between April and July 1887. The purpose of this experiment is to prove the existence of ether. This hypothetical medium permeating space was thought to be the carrier of light waves. This experiment aimed to measure the speed of light in two ...
The definitive experiment was carried out by Alvager et al., Physics Letters 12, 260 (1964). LIGO. We have to mention here that the most spectacular realization (so far) of Michelson's interference techniques is the successful detection (first in 2016) of gravitational waves by a scaled-up version of the interferometer.
Albert Michelson and Edward Morley set out to detect this aether. Although Einstein himself did not consider the results of Michelson and Morley's experiment as necessary for his development of Theory of Relativity, the experiment is nonetheless important that indirectly supported both Maxwell's theories and the postulates that underpin Einstein's.
The Michelson Morley Experiment was one of the failed experiments that stands as proof against the existence of the luminiferous ether concept. Michelson and Morley tried to explain that Earth moved around the sun on its orbit, and the flow of substances like ether across the Earth's surface could produce a detectable "ether wind".
The most famous and successful was the one now known as the Michelson-Morley experiment, performed by Albert Michelson (1852-1931) and Edward Morley (1838-1923) in 1887. Michelson and Morley built a Michelson interferometer, which essentially consists of a light source, a half-silvered glass plate, two mirrors, and a telescope.
The MICHELSON-MORLEY EXPERIMENT was performed in the basement of a WESTERN RESERVE UNIV. dormitory in July 1887 by ALBERT A. MICHELSON of the Case School of Applied Science and EDWARD W. MORLEY of Western Reserve Univ. it was designed to detect the motion of the earth through the "luminiferous aether," a theoretical substance which, according to 19th century physicists, was essential to the ...
Michelson, with the collaboration of E. W. Morley, constructed a new interferometer with multiple mirrors and a pathlength about 10 times longer. This device should have given a fringe shift of about 0.4, but they observed less than 0.005 fringe (A. A. Michelson and E. W. Morley, Am. J. Sci., 134, 333 (1887)).